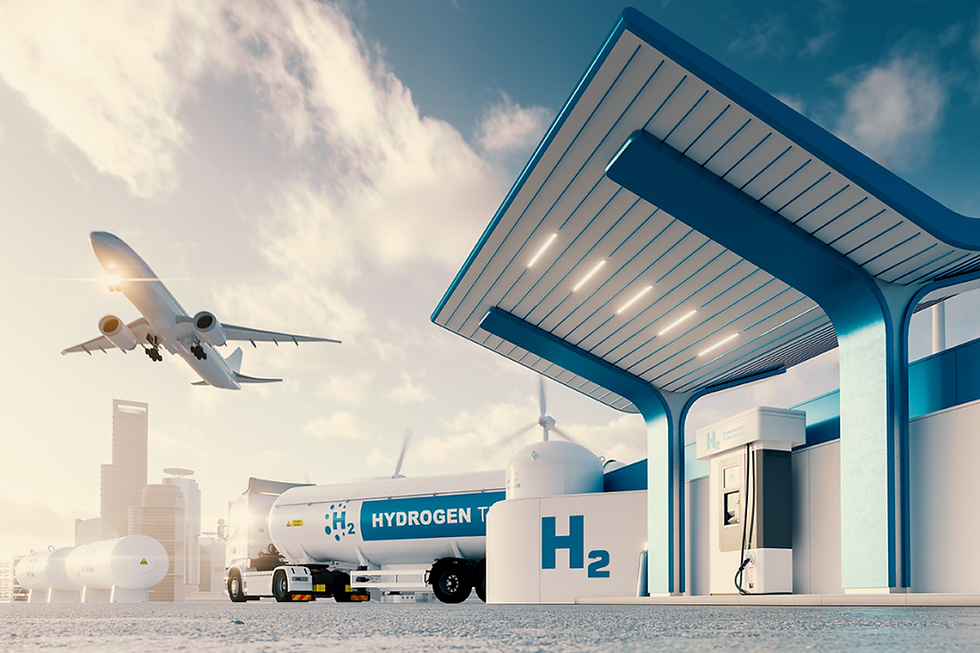
Hydrogen fuel cell electric vehicles (FCEVs) have been in and out of the spotlight several times over the last two decades. In fact, the first FCEV (the Chevy Electrovan) dates back to 1966, while more practical examples were introduced in the early 2000s in low volumes by nearly every major automaker as concepts. In 2008, Honda was the first to lease an FCEV to actual customers, while Hyundai was the first automaker to truly mass produce an FCEV, beginning with the ix35 Fuel Cell in 2013. In all cases, FCEV uptake has been very limited, making up just 10,000 sales per year, typically in specific markets such as South Korea, Tokyo, or California, where hydrogen fueling networks are best-established. So why would a customer want an FCEV instead of a BEV (or ICE for that matter). The promise of the FCEV is that it provides the green mobility of a BEV, emitting only water from the tailpipe, yet has the refueling convenience of an ICE vehicle, requiring just 3-5 minutes to refuel and providing a long range on a full tank. It’s a promise of the best of both worlds, and from the consumer’s perspective, it just might be. As always though, the devil is in the details.
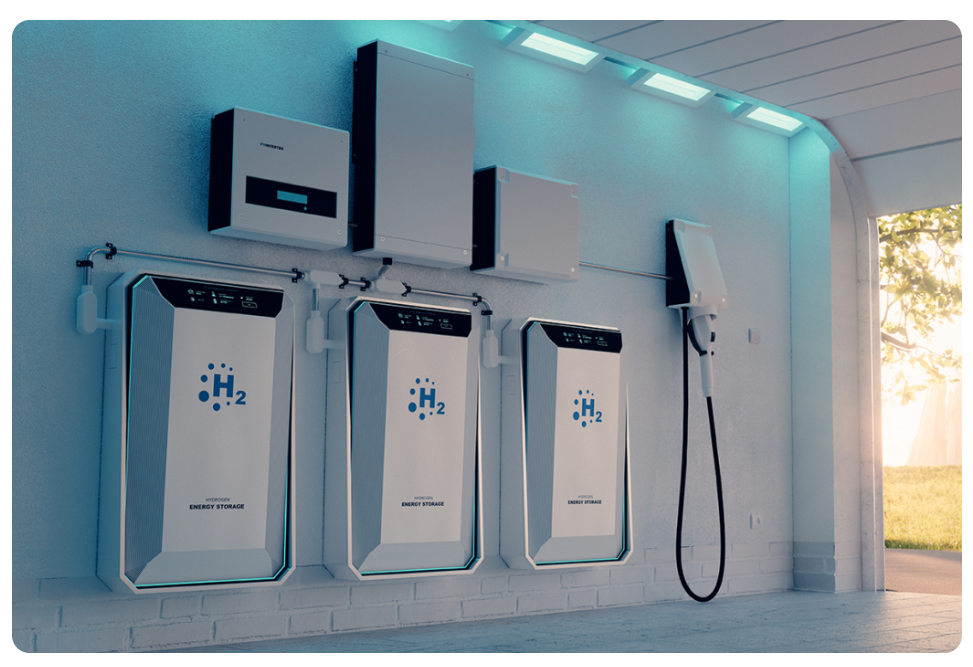
In the previous article on this topic, we talked about how BEVs actually have a very large initial carbon footprint compared to their ICE counterparts. This is a critical measure for any vehicle and it helps explain why many companies and governments see BEV as just a stepping stone to something better. Fuel cell technology may indeed be that ‘something better’, but let’s look at the cradle-to-grave details first. Current estimates show that FCEV production and recycling emits about 40% as much greenhouse gas as BEVs. The carbon footprint of BEVs is not only a concern for automakers and environmental protection groups, but also for consumers. In fact, in SBD’s 2021 survey of over 6,000 consumers, 38% of ICE owners were concerned about the environmental impact of battery production, compared to 69% of EV owners, showing that awareness of carbon footprint is likely to increase as adoption of EVs increases. So right from the start, it may seem that FCEVs would be the obvious path forward compared to BEV, but of course we have to look at the use phase as well. This is where things get more complicated.
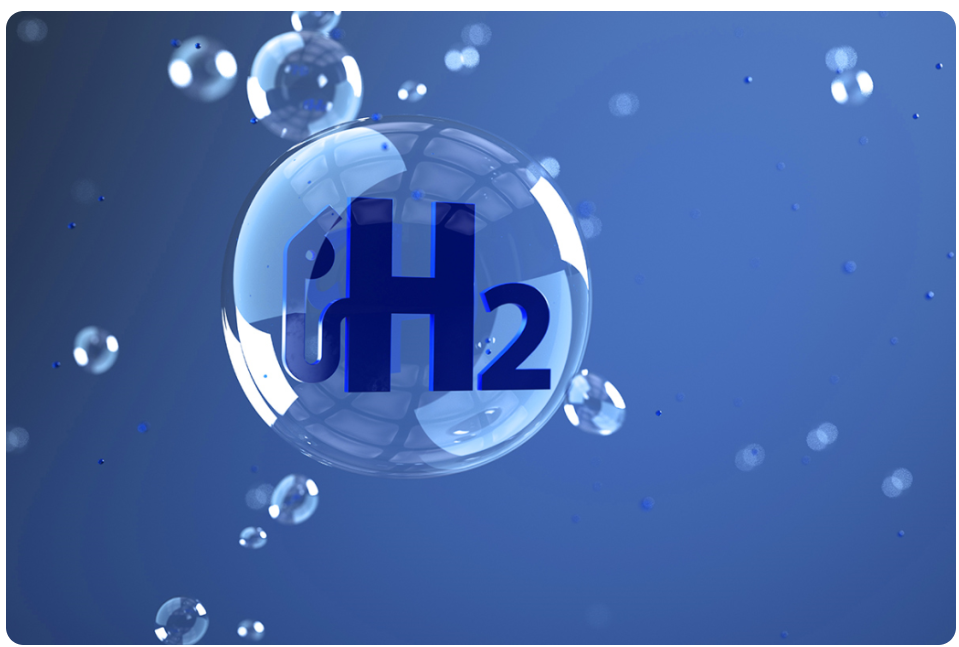
First, let’s look at the fuel itself. Hydrogen can be produced in a variety of ways. Because it is a very lightweight gas in its natural form as H2, it does not exist in significant quantities in the lower atmosphere, meaning that we can’t simply collect it from the air. Instead, we have to extract it from a molecule where the hydrogen is bonded to other elements. These molecular bonds are very strong and require a lot of energy to break. The most abundant source of hydrogen is simply water where two hydrogen atoms are bound to one oxygen atom as H2O. A simple method for separating these elements is through the process of electrolysis where an electric current is passed through the water, causing the molecular bond to break and causing the elements to be released as a gas. This method of producing hydrogen gas is very flexible in that any source of electricity can be used, allowing producers to choose fully renewable sources such as solar and wind power. When renewable sources are used, the hydrogen is referred to as ‘green’ hydrogen.
Electrolysis, however, is not the most efficient method for producing hydrogen, with efficiencies topping out around 80% and costs around 10 USD/kg. The vast majority of hydrogen produced today (around 96%) comes from steam reformation of methane (natural gas) which can be up to 85% efficient and costs only around 2 USD/kg. Hydrogen gas produced in this way, as well as from other hydrocarbon feedstocks, is referred to as ‘blue’ hydrogen. Because blue hydrogen comes from hydrocarbons, it is non-renewable, and without carbon-capture processes, it may also release significant quantities of carbon into the atmosphere in the form of carbon monoxide and dioxide. It’s because of this negative environmental impact that it’s critical for FCEVs to use only certified green hydrogen to realize the environmental benefits that they offer. With a price currently five times that of blue hydrogen, commercial success will only be achieved through government mandates and consumer consciousness of the effects of hydrogen production.
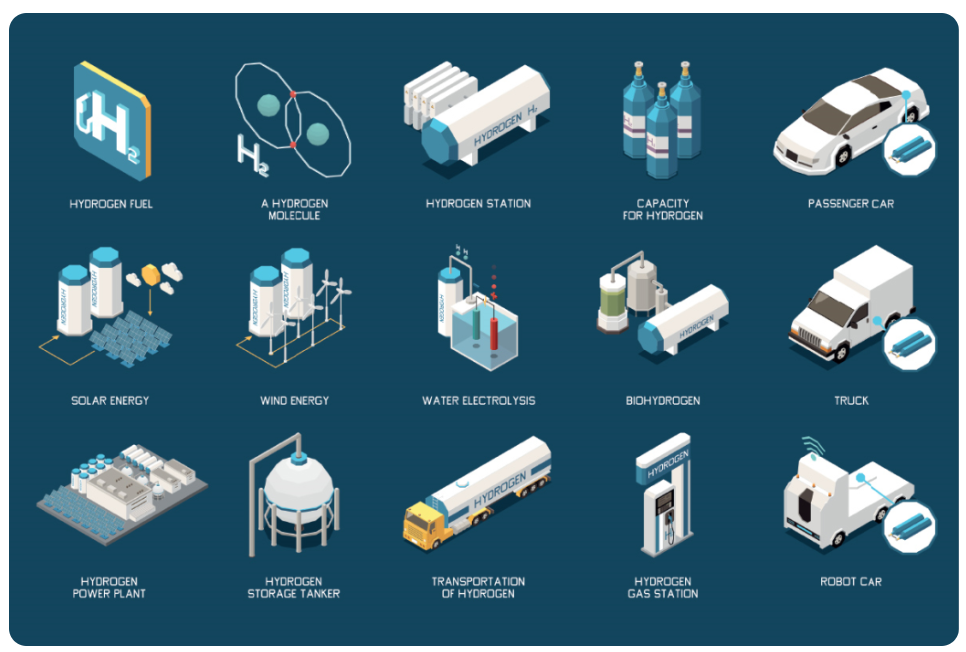
Once the hydrogen is produced, it must be transported to a filling station. In the future, long distance transport could be primarily through pipelines, but currently, most of the transportation is performed by refrigerated trucks. Of course the transportation and refrigeration also requires energy, which varies based on the specific location. Once transported to the filling station, it needs to be stored in the vehicle’s hydrogen tank. Because the density of hydrogen is so low (0.07 g/L), the gas must be compressed to extreme pressures for storage in the vehicle. As an example, the Toyota Mirai can hold approximately five kgs of hydrogen in its tanks. If the gas were at standard atmospheric pressure, the tank would need to have a 71,500 liter volume, which of course is impractical. Instead, the Mirai’s tank is only 142 liters, so the hydrogen must be compressed to 700 bar (more than 10,000 psi) to fit those five kgs of hydrogen into the tanks. This compression process also consumes energy and generates waste heat at a cost of about 10% of the energy contained in the hydrogen.
When used to power FCEVs, hydrogen can be thought of as a chemical carrier of electricity, since electricity is essentially ‘converted’ into hydrogen at the electrolysis plant and then back into electricity in the vehicle’s fuel cell. Unfortunately, this conversion on both ends erodes the efficiency of the overall process significantly. Modern fuel cells are around 60% efficient, meaning that 40% of the energy gained from hydrogen and oxygen recombination is in the form of heat, not electricity. In cold climates, this heat energy can be used to warm the cabin of the vehicle, dramatically improving the system efficiency compared to a BEV which must use electricity to heat the cabin, thereby depleting driving range. In warm climates however, the heat is simply dissipated to the atmosphere, bringing us back to the 60% efficiency.
The rest of the process is nearly identical between FCEVs and BEVs: the electricity produced by the fuel cell or battery is then converted into an alternating waveform to power the motors in the car’s powertrain. FCEVs typically contain a small battery that allows them to recover kinetic energy during braking just like a BEV, making the driving efficiencies roughly the same. But if we step back and compare the ‘well-to-wheels’ efficiency figures of a BEV and comparable FCEV, you can imagine that the FCEV is not looking very promising at this point. Even if both energy streams were powered by the same renewable energy, the FCEV ends up being around 40% efficient while the BEV is closer to 80% efficient, owing primarily to loses in electricity transmission and AC to DC conversion.
So with an efficiency roughly half that of a BEV, why are we even considering FCEVs? As we discussed earlier, the production of FCEVs is more environmentally friendly than BEVs, making FCEVs very attractive to OEMs and governments. In addition, although the efficiency is much lower, the fuel for FCEVs can be produced entirely with renewable energy, so looking good in the eyes of environmental regulators as well. Yet the real promise for FCEVs is not with cars at all, but rather long haul trucks. As we discussed in the first post in this series, long haul trucks are a poor candidate for battery-powered propulsion due to the enormous quantities of batteries required which reduces the maximum payload and requires lengthy charging times. Because compressed hydrogen is extremely energy-dense, a fuel-cell-powered truck would reap the same benefits as a fuel-cell-powered car: fast refueling, long range, and a low production carbon footprint. The efficiency problems are the same as well, however the efficiency of a fuel cell is still greater than that of a diesel engine, making hydrogen possibly the only option for a carbon-neutral long haul truck fleet with today’s technology.

Many governments are starting to see hydrogen not just as a fuel, but as an entire economy, potentially forming the backbone of energy storage and transmission and enabling energy independence where it may not have been feasible before. Hydrogen is also quite versatile since it can be burned as a clean fuel or used as the basis for production of other fuels such as ammonia. Because of these factors, there is strong government support for hydrogen research and infrastructure development, increasingly from France, Germany, Japan, and South Korea. If researchers are able to significantly improve the efficiency of electrolyzers and fuel cells, FCEVs may well become the most environmentally-friendly option for personal transportation, but we have a long way to go. Another possibility is the development of denser forms of hydrogen that can be used without extreme compression. For instance, Ammonia (NH3) can be produced from hydrogen and atmospheric nitrogen. It contains around 1600 times as much hydrogen for the same volume at atmospheric pressure compared to hydrogen. There are still many issues to be resolved with the ammonia fuel cell process, yet with the right advances, it could enable very high energy densities and rapid refueling, while supporting carbon-neutral transportation and fitting in very nicely with a hydrogen-based economy.
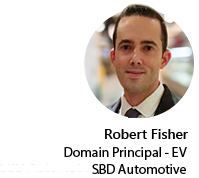